Microscopy
Dynamic Optics and Photonics
Microscopy
The DOP group undertakes significant research into the development and improvement of optical microscopes, with applications ranging from biological and medical imaging to industrial inspection.
There are many types of optical microscope, which use different optical systems such as widefield, laser scanning, and parallelised scanning systems. Our research spans across many microscope modalities, encompassing improvements in resolution, image quality, cost and size of the system. A particular focus of our work is the development of adaptive optics for aberration correction in microscopes.
Biological Microscopy
Many applications of our high resolution microscopes are in the biological sciences. Our research includes advances in the technology of confocal and two-photon fluorescence microscopes, harmonic generation microscopes and other high resolution methods.
Applications have included cell biology, developmental biology and neuroscience. The group is currently working closely with the Lak group and Bruno group in the Department of Physiology, Anatomy and Genetics (DPAG) at the University of Oxford, and the Silver lab at University College London to study neural circuits in the brain.
Examples of images taken with high resolution microscopes using DOP group technologies.
Publications
Universal adaptive optics for microscopy through embedded neural network control
Qi Hu, Martin Hailstone, Jingyu Wang, Matthew Wincott, Danail Stoychev, Huriye Atilgan, Dalia Gala, Tai Chaiamarit, Richard M. Parton, Jacopo Antonello, Adam M. Packer, Ilan Davis & Martin J. Booth. Light: Science & Applications 12: 270 (2023)
CryoSIM: super-resolution 3D structured illumination cryogenic fluorescence microscopy for correlated ultrastructural imaging
Michael A. Phillips, Maria Harkiolaki, David Miguel Susano Pinto, Richard M. Parton, Ana Palanca, Manuel Garcia-Moreno, Ilias Kounatidis, John W. Sedat, David I. Stuart, Alfredo Castello, Martin J. Booth, Ilan Davis, and Ian M. Dobbie. Optica 7(7) 802-812 (2020)
Ultra-High Resolution 3D Imaging of Whole Cells
Fang Huang, George Sirinakis, Edward S. Allgeyer, Lena K. Schroeder, Whitney C. Duim, Emil B. Kromann, Thomy Phan, Felix E. Rivera-Molina, Jordan R. Myers, Irnov Irnov, Mark Lessard, Yongdeng Zhang, Mary Ann Handel, Christine Jacobs-Wagner, C. Patrick Lusk, James E. Rothman, Derek Toomre, Martin J. Booth & Joerg Bewersdorf. Cell 166 (4), 1028–1040 (2016).
Super resolution microscopy
Super-resolution microscopes enable the visualisation of objects much smaller than the physical diffraction limit of light, which is in the hundreds of nanometres range. They are widely used in biological applications to resolve features in the tens of nanometres range, such as sub-cellular structures. Super-resolution microscopes are particularly sensitive to aberrations in the optical system, so adaptive optics has been particularly important in this application.
The group is developing custom super-resolution techniques, including 4Pi-single molecule switching (4Pi-SMS) microscopes and Structured Illumination microscopes (SIM). Work is carried out in collaboration with the Micron imaging facility in the Department of Biochemistry at the University of Oxford.
Publications
Implementation of a 4Pi-SMS super-resolution microscope
Jingyu Wang, Edward S. Allgeyer, George Sirinakis, Yongdeng Zhang, Kevin Hu, Mark D. Lessard, Yiming Li, Robin Diekmann, Michael A. Phillips, Ian M. Dobbie, Jonas Ries, Martin J. Booth & Joerg Bewersdorf. Nature Protocols 16, 677–727 (2021)
Deep super-resolution imaging of thick tissue using structured illumination with adaptive optics
Jingyu Wang, Danail Stoychev, Mick Phillips, David Miguel Susano Pinto, Richard M. Parton, Nick Hall, Josh Titlow, Ana Rita Faria, Matthew Wincott, Dalia Gala, Andreas Gerondopoulos, Niloufer Irani, Ian Dobbie, Lothar Schermelleh, Martin Booth & Ilan Davis. bioRxiv (2023) doi:10.1101/2023.12.21.572818
IsoSense: frequency enhanced sensorless adaptive optics through structured illumination
Mantas Žurauskas, Ian M. Dobbie, Richard M. Parton, Mick A. Phillips, Antonia Göhler, Ilan Davis & Martin J. Booth. Optica 6, 370-379 (2019)
Three-dimensional adaptive optical nanoscopy for thick specimen imaging at sub-50-nm resolution
Xiang Hao, Edward S. Allgeyer, Dong-Ryoung Lee, Jacopo Antonello, Katherine Watters, Julianne A. Gerdes, Lena K. Schroeder, Francesca Bottanelli, Jiaxi Zhao, Phylicia Kidd, Mark D. Lessard, James E. Rothman, Lynn Cooley, Thomas Biederer, Martin J. Booth & Joerg Bewersdorf. Nature Methods 18(6), 688-693 (2021)
Remote Focussing Microscopy
Adaptive optics (AO) techniques have applications beyond the correction of optical aberrations. They can also be used to refocus rapidly inside specimens without any mechanical movement of the specimen or imaging instruments.
This feature is especially valuable for 3D imaging in live animals, and in cases where motion of the specimen would be undesirable, for example, when using electrophysiology electrodes or microinjection needles. It is also useful for obtaining depth information in 3D samples at high speed and with minimal aberration, such as for volumetric imaging in biological samples, and precision manufacturing in industrial materials. Conventional methods such as translation of the objective lens or sample stage suffer from inertia, and imaging using high-numerical aperture lenses introduces high-order aberration modes when focusing into refractive index-mismatched media.
The group is developing fast and robust remote-focussing algorithms using dynamic devices, such as deformable mirrors and spatial light modulators, to provide aberration-free imaging depth translation by deforming the wavefront. AO devices are used to generate defocus patterns to modulate the phase of the incident or emitted light. The defocus patterns can be updated in a speedy and motion-less manner, typically over 1KHz. This process may introduce high-order aberrations, but AO-based devices can be used to correct for residual optical aberrations at the same time.
Schematic showing deformable mirror-based remote focusing methods.
This technique is extremely valuable for super-resolution imaging, and also has applications in deep brain neuroimaging, clinical imaging, and precision laser fabrication. Work is carried out in collaboration with the Emptage Group in the Department of Pharmacology at the University of Oxford, the Lak group and Bruno group in the Department of Physiology, Anatomy and Genetics (DPAG) at the University of Oxford, and the Silver lab at University College London.
Deformable mirror-based remote focusing methods used in live specimen imaging, showing microtubules in a COS-7 cell.
Publications
Extended range and aberration-free autofocusing via remote focusing and sequence-dependent learning
Jiahe Cui, Raphaël Turcotte, Nigel J. Emptage & Martin J. Booth. Opt. Express 29, 36660-36674 (2021)
Generalised adaptive optics method for high-NA aberration-free refocusing in refractive-index-mismatched media
Jiahe Cui, Jacopo Antonello, Andrew R. Kirkpatrick, Patrick S. Salter & Martin J. Booth, Opt. Express 30, 11809-11824 (2022)
Aberration-free three-dimensional multiphoton imaging of neuronal activity at kHz rates
Edward J. Botcherby, Christopher W. Smith, Michael M. Kohl, Delphine Débarre, Martin J. Booth, Rimas Juškaitis, Ole Paulsen, and Tony Wilson. Proceedings of the National Academy of Sciences 109, 2919-2924 (2012).
Microscopy For Manufacturing
Many manufacturing tasks require accurate optical imaging to provide feedback for process control, thereby increasing efficiency and reducing wastage. The group develops a range of optical imaging techniques that are appropriate for integration into future (or existing) manufacturing systems. These give real-time information on the position of the workpiece or the status of the manufacturing task.
These methods include microscope modalities tailored to the manufacturing task. For example, inline phase microscopy measures changes in refractive index due to laser writing; polarisation microscopy can monitor the creation of nanoscale structures in materials; confocal microscopy is used to detect material changes through fluorescence or Raman signals.
The group tests and develops new technologies in conjunction with research on Laser-based manufacturing, and works with external partners in industry or through the Future Metrology for Manufacturing hub at the University of Huddersfield.
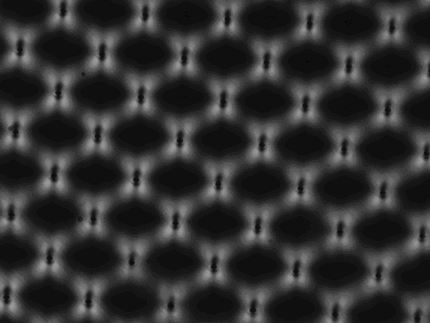
A cross-polarised microscope image displaying the strain field
around an array of laser written contacts in diamond.
Publications
Tomographic refractive index profiling of direct laser written waveguides
Nicolas Barré, Ravi Shivaraman, Lisa Ackermann, Simon Moser, Michael Schmidt, Patrick Salter, Martin Booth & Alexander Jesacher Opt. Express 29, 35414-35425 (2021)
Microscope calibration using laser written fluorescence
Alexander D. Corbett, Michael Shaw, Andrew Yacoot, Andrew Jefferson, Lothar Schermelleh, Tony Wilson, Martin Booth, and Patrick S. Salter Opt. Express 26, 21887-21899 (2018)
Laser writing of individual nitrogen-vacancy defects in diamond with near-unity yield
Yu-Chen Chen, Benjamin Griffiths, Laiyi Weng, Shannon S. Nicley, Shazeaa N. Ishmael, Yashna Lekhai, Sam Johnson, Colin J. Stephen, Ben L. Green, Gavin W. Morley, Mark E. Newton, Martin J. Booth, Patrick S. Salter, and Jason M. Smith Optica 6, 662-667 (2019)
Microscopy Group Members
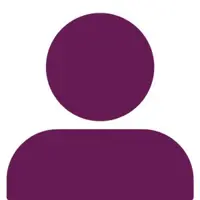
Jingyu Wang
Postdoctoral Research Assistant
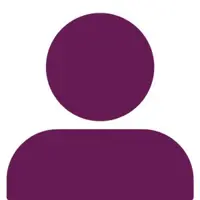
Alvaro Fernandez Galiana
Postdoctoral Research Assistant
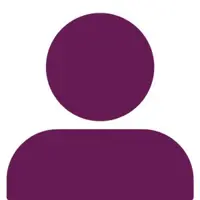
Biwei Zhang
DPhil Candidate