29 Apr 2021
Nanomaterials engineered for high-speed impact
Engineers from the universities of Oxford, Birmingham and Ghent have demonstrated a fundamentally new energy absorption mechanism under high-speed mechanical impact
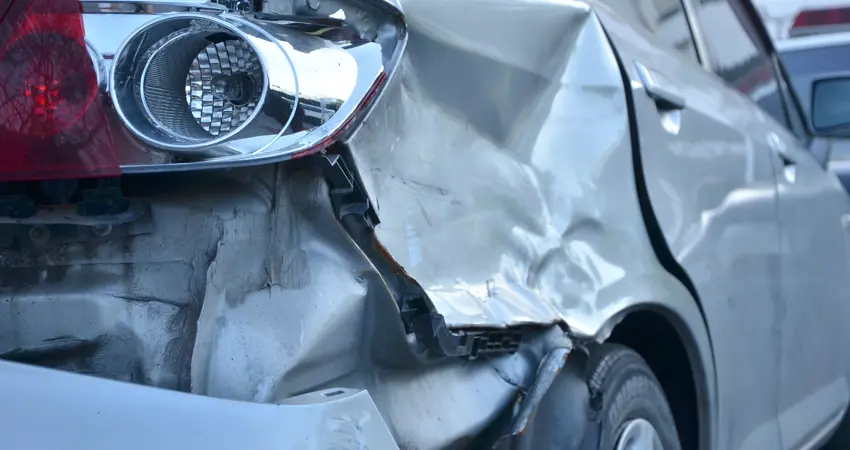
Crumple zones in automobiles, trains, and other large vehicles are commonly made from metals, which will permanently deform, crush and collapse during a collision
Efficient absorption and dissipation of a sudden surge of mechanical energy generated by a high-speed collision underpin the design and engineering of modern protection technologies. For example, the crumple zones in automobiles, trains, and other large vehicles are commonly made from metals, which will permanently deform, crush and collapse during a collision. This mechanism absorbs impact energy and reduces the abrupt deceleration of passengers inside the vehicle, thereby cushioning the blow to minimise physical trauma. Lightweight porous materials such as metal foams, layered composites, and honeycomb structures have also been deployed for impact protection.
Recently, an international team of engineers from the universities of Oxford, Birmingham and Ghent have demonstrated a fundamentally new energy absorption mechanism under high-speed mechanical impact, reported in the journal Nature Materials.
In contrast to other state-of-the-art impact attenuating materials, they show a major enhancement in energy absorption capacity combined with excellent material reusability. These two material performance criteria cannot be met concurrently by conventional materials.
The study employs hydrophobic (water repelling) framework nanocrystals, constructed from a periodic array of cage-type porosity (FIG.1). The dimension of a nanocage is only about 1 nanometre (1 nm = one billionth of a metre). Adjacent pores are interconnected by very narrow apertures, whose size is comparable to that of a single water molecule (0.3 nm).
FIG.1: (Left) Electron microscope image of the water-repelling crystals. (Right) Nanocages present within a single crystal, comprising interconnected pores (yellow surfaces) to afford water intrusion and extrusion.
Using pressurised intrusion of liquid water into the hydrophobic nanopores, they measured the energy absorbed under realistic conditions pertinent to real-world applications (FIG.2).
"We found that under a high-speed impact the energy absorption capacity is enhanced almost 20-fold compared with a slow compression. This strong rate-dependent mechanical response of hydrophobic nanocages is yet unknown in the field", says Professor Jin-Chong Tan from Oxford's Multifunctional Materials & Composite (MMC) Lab, who leads the study.
"Remarkably, the cyclic tests further revealed that the nanoporous framework is mechanically resilient. It could withstand at least 1,000 intrusion-extrusion cycles without material degradation", he adds.
FIG.2: Contrasting the energy absorbed by the nanoporous framework material (shaded area under the curves) subject to a high-speed impact relative to a slow compression.
To understand the underpinning mechanisms, they employed computational modelling to shed light on the physical and chemical processes involved. Molecular dynamics simulations performed at the University of Ghent revealed that the observed rate effect stems from the intrinsic nanosecond timescale to yield stable water clusters in hydrophobic nanocages. This mechanism controls water transport across the array of nanopores.
"This research shows how, by working with colleagues in other engineering disciplines, we can create unique opportunities to make significant advances in the materials that we can use for these challenging applications", says Professor Clive Siviour from Oxford Engineering Science's High Strain Rate Group, a co-author of the paper.
"Crucially, our research has unlocked a new dimension to understanding the mechanics of nanoporous materials, where the adaptive energy absorption by hydrophobic frameworks can be exploited for commercial use", comments Professor Tan.
"The findings could pave the way to numerous real-world applications, stimulating the design of high-performance impact protection systems for peoples’ safety and comfort in the engineering, industrial and consumer sectors."
The research was supported by an ERC Consolidator Grant (PROMOFS) and a KC Wong Fellowship.
Read the full paper, ‘High-rate nanofluidic energy absorption in porous zeolitic frameworks’ in Nature Materials.